The history and science of nuclear energy and the climate problem, part 1: why solar and wind energy is insufficient
In this article, Nowa Ammerlaan argues that focusing solely on solar panels and wind turbines, as all left-wing parties are currently doing, is insufficient and unrealistic, and therefore advocates the use of, among other things, nuclear (fission) energy.
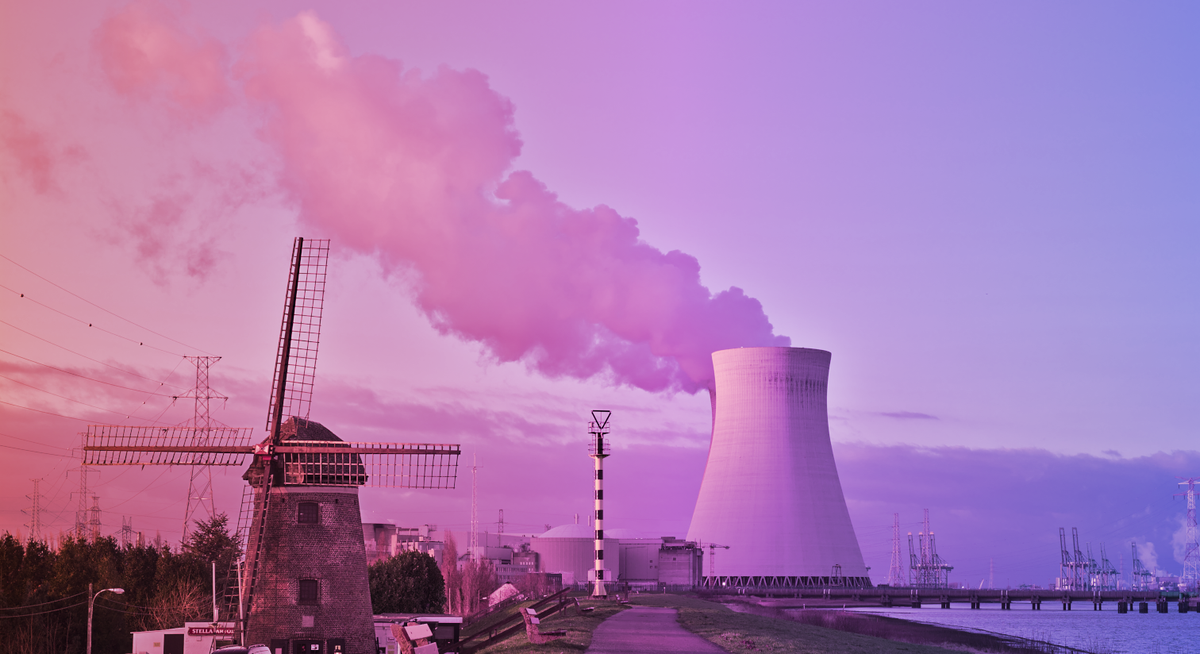
This is a loosely translated version of the article series “De Kern van het Klimaatprobleem” originally written by Nowa Ammerlaan in 2020 and published on the website of the Dutch Communist Platform.
The year is 2021, almost 5 years after the signing of the Paris climate agreement, an ambitious accord to limit global warming to 1.5°C. In the meantime the earth has already warmed up more than 1.1°C compared to the ‘pre-industrial’ era (1850-1900). And so far, this curve does not show any signs of flattening any time soon. The fight for socialism is the fight for the future, and thus also the fight against climate change, but how do we fight climate change effectively? In this article I will argue that limiting this battle to the use of solar panels and wind turbines, which is the predominant strategy across the entire left, is wholly insufficient and completely unrealistic. I therefore advocate to complement this strategy with the use of other energy sources, among which nuclear fission energy.
Part 1: Why solar and wind energy is insufficient
Development and limitations of solar panels and wind turbines
I will start this first part by analysing both the current state of solar and wind technologies, and how we can expect these technologies to improve in the future. I will continue with talking about the storage of electricity. And conclude with arguing for an electricity grid which is as diverse as possible.
The not-so-unlimited potential of Silicon
The efficiency of Silicon solar cells has been steadily increasing over the years. However, it would be incorrect to assume that this trend will continue on in the future. All good things must come to an end, and as all too often thermodynamics is the reason we can’t have nice things.
The efficiency of any solar cell is thermodynamically limited by the Shockley-Queisser limit, for Silicon solar cells (read: all commercially available solar cells) this happens to be 29.43%[1]. Of course nothing is perfect, and in practice the actual efficiency is always lower than this limit. Modern commercially available solar cells typically have an efficiency of around 24%, and thus are already very close to their fundamental limit. This is why the developments in the efficiency of solar cells is stalling.
So how do we continue improving the solar cell? Luckily, it is still possible to reach higher efficiencies, we just have to choose a different material! Independently of the chosen material the maximum efficiency has been determined to be 33.77%[2]. Therefore, by choosing a different material whose Shockley-Queisser limit is closer to this theoretical maximum of 33.77% we can still find some room for improvement.
High-efficiency solar cells are already used in areas where the energy output per unit of mass is more important than the production costs, such as in satellites and other spacecraft. Gallium Arsenide holds the world record for highest efficiency single-junction solar cell with a whopping 29.1% and is therefore the material of choice for such applications.
A second way to increase the efficiency of solar cells is to combine multiple single-junction solar cells of a different material into one multi-junction solar cell. Each individual junction absorbs a different part of the solar spectrum, and is still bound by their respective Shockley-Queisser limit. However, the combination of these single-junctions is not. A promising candidate for a tandem (=two-junction) solar cell is the combination of Silicon with a perovskite material. Where perovskite refers to the crystal structure of this group of materials. Usually, such a material is a combination of an organic and an inorganic material, where the latter is often Lead or Tin joined with a halide (the halides are the elements in the second rightmost column of the periodic table: Fluorine, Chlorine, Bromine, Iodine and Astatine). Such a tandem solar cell has already reached efficiencies of 29.1% in practice. Furthermore, the overall world record for solar cell efficiency was set at 47.1% in 2019 by a solar cell consisting of six different junctions[3].

Figure 1: Developments in the efficiency of solar cells over the years
As we have seen, the answer to the question ‘can we make higher efficiency solar panels?’can be answered with a resounding yes! However, this is not the right question to ask, because producing a solar panel only makes sense if it will produce more electricity in its lifetime than was consumed in its production process. Silicon based solar panels have the huge advantage that they are a) relatively easy to produce, and that b) Silicon is a very common element on earth, which is relatively easy to extract. As a general rule of thumb, the more complex (e.g. more junctions) you make the solar cell, the more electricity it will cost to produce it. And at some point the efficiency gained by the increased complexity is no longer worth it.
Up to now we’ve been getting away with improving the existing technology of Silicon solar cells, however as we have seen this route is reaching a dead-end. To continue improving solar cells we have to use more exotic materials, which of course greatly increase the amount of electricity consumed in the production of the solar cell. The right question therefore should be ‘can we make viable higher efficiency solar panels?’, the answer to which regrettably is no at the moment.
Therefore, it is to be expected that the trend of increasing efficiency that we have seen in commercially available solar panels in the past, will stall and remain stalled for the foreseeable future. At least until some brilliant new breakthrough will greatly reduce the amount of electricity consumed in the production of multi-junction solar cells. All too often I see people pointing at this past trend of increasing efficiency, mistakenly expecting this trend to continue, and then concluding that some super-high efficiency solar panel will come to our rescue and aid us in the energy transition. It is good to hope, but we cannot base policy on such hope. We should stay realistic, and therefore assume that we will have to make do with the current Silicon solar cell technology in the energy transition.
How far can these solar panels get us? A study conducted in 2019 estimates that about 25% of the energy consumption in the European Union can be generated using rooftop solar panels alone[4]! Considering that in that same year only 4% of the electricity produced in the EU was solar, there is still a lot of room for more solar energy. However, as we shall see a theoretical possibility does not necessarily equate to a realistic, practical or timely solution, nor does the problem stop at generating the electricity, we also need to store and transport it.
The wind in the sails of the energy transition?
At first glance one might think that the workings of a wind turbine are relatively easy. However, the problem very quickly becomes a lot more complicated once we aim to maximize the efficiency, and start to consider the effect that one wind turbine has on a neighbouring one in a wind farm. There are an incredible number of factors that affect the efficiency of a wind turbine, such as the shape and size of the blades, the strength but also stability of the wind, the losses incurred in the transmission mechanism and the generator, and also the shape and amount of obstacles in the vicinity, such as other turbines[5].
The theoretical maximal attainable efficiency of any wind turbine is given Betz’s law. This law is based on the principle of conservation of mass and momentum, obviously the total amount of air-mass entering the turbine area should equal the amount of air-mass exciting this area on the opposite side. An equally obvious statement is that a turbine can never extract 100% of the energy contained in the wind, if it would the turbine would cause the wind to stop and air would accumulate just behind the turbine. This of course is completely unphysical, for the turbine to work it must be supplied with new air blowing in from the front, and therefore the air at the back of the turbine must make way. As a consequence there will always be some energy left in the wind after it exits the turbine. Betz’s law therefore concludes that no more than 59.3% of the energy contained in the wind can be extracted. Of course reality is not perfect, and factors such as friction cause energy to be lost. In practice a modern wind turbine has a peak-efficiency of around 44.5% to 47.4%.

Figure 2: Vattenfall. Photographer is Christian Steiness.[6]
Wind energy realises its true potential at sea, where the wind is stronger, more constant, and more stable. Under such conditions a wind turbine can be up to 16% more efficient[7]! Additionally, wind turbines do make some noise (about 45dB at a distance of 300 meters, which is slightly louder than a refrigerator this, together with the flickering shadow they produce, can become an annoyance to residents living very close to it. Such concerns are nullified at sea.
Combining multiple wind turbines into one big wind farm is advantageous in terms of energy transport. Since this would require one big cable instead of multiple smaller ones, especially for offshore turbines this obviously makes things a lot easier. The disadvantage of placing wind turbines close to one another is that the turbines will be, to some extent, in each other’s ‘wind shadow’, because once the wind has passed through one turbine it will naturally be weaker and more turbulent when it hits a second turbine. This turbulence can reduce the efficiency of wind turbines in the middle of a wind farm by as much as 20% compared to the turbines at the edges of the wind farm[8]. On the 12th of February 2008 rare weather conditions in the North Sea near Denmark caused this ‘wind shadow’ phenomena to be visualized by the mist forming in the wake of the wind turbines of the Horns Rev 1 wind farm, as can be seen in Figure 2[9].
In 2009 a report from the European Environmental Agency claimed that there was enough usable wind, land and sea to meet the energy demand of the entire European Union ten times over. While this study does not seem to take into account the effect that neighbouring wind farms have on each other, an effect which will most certainly become relevant when implementing wind energy on this massive scale[10], this does show us the enormous potential that wind energy has, especially considering that in 2017 only 11.6% of the electricity used in the European Union had been generated using wind energy.
Storage and the importance of a stable, robust, flexible and diverse electricity grid
“What to do when the sun doesn’t shine and the wind doesn’t blow” is the classic objection raised against solar and wind energy, especially the climate-change-denying right is very fond of using this argument to depict plans for combating climate change as unrealistic. However, it would be foolish to just discard this very real concern, merely because it was raised by reactionaries and the defenders of the vested interests of the fossil industries. At first glance the solution to this predicament seems obvious, simply store the generated energy when more is generated than is used for use on a rainy day. Sadly this is more easily said than done.
Electricity, or more accurately: electrical energy, itself cannot be directly stored, it has to be converted to some other form of (potential) energy which can be stored. This can for example be done using batteries (chemical energy), flywheels (mechanical energy), pumped-(water)-storage (gravitational energy), or by using electricity to create some kind of fuel such as Hydrogen (from water) or Methane (from water and carbon dioxide).
Each storage technology has their own distinct advantages and disadvantages. However, they all have one thing in common: losses. Once again the party is ruined by the usual suspects: thermodynamics, friction and resistance. Any conversion of energy from one form to another will always cause energy to be lost, and any form of electricity storage will have at least two such conversions (from electricity, and back to electricity). Which means that storing electricity will inherently cause some of it to be lost, it is therefore always better to use it for something useful directly instead. Exactly how much electricity is lost depends on the storage technology that is used, it can be as little as 5% or as much as 60%.
How much storage would we need? Of course, the more variable energy sources (=energy sources depending on factors outside of human control such as the weather), the more storage capacity would be required to compensate for the downtime of those sources. And in turn, the more storage capacity there is, the more additional energy sources required to actually fill this storage. A 2018 study shows us that depending on the ratio solar to wind energy, about 15% to 140% storage capacity is required to fully transition to only wind and solar energy[11]. Compared to wind energy, solar energy requires significantly more short term energy storage to keep the grid stable. And as such the 140% represents the scenario where the energy supply is almost entirely solar, and the 15% represents the opposite scenario where the energy supply is almost entirely wind. That being said, this study emphasizes the large uncertainty in such predictions due to their dependency on the flexibility and (local) transport capacity of the energy grid.
Diversity, flexibility, sustainability and robustness.
Can we provide the entire European Union with electricity using only solar, wind, and the therefore required storage capacity? As we have seen, yes, in theory we can! However, this is only part of the question. Can we reform the entire grid in this way in time to prevent total climate catastrophe? Can we mine and process all the required resources for the enormous amount of solar panels, wind turbines and storage? Does this strategy result in the most stable, most robust, most flexible energy grid? Is this strategy the most sustainable, or will it just cause another different crisis? In short, just because we can, should we disregard everything else and throw all our efforts into only solar and wind? Will this lead to the desired result of a stable, robust, sustainable energy grid?
The answers to these questions are often left unanswered. Authors often show that we can, and presume that this is enough and also means that we should. A 2018 report by the Dutch Ministry of Infrastructure and Water Management highlights the difficulties in meeting the exploding demand in rare-earth elements, and concludes that to avoid scarcity we must either reduce the consumption of these elements, redesign existing technologies to allow for way more recycling, or open new (European) mines. And I haven’t even started about the abhorrent conditions in Congolese Cobalt mines, which are still the major supplier of Cobalt, an essential component in batteries.
Almost all countries are way behind schedule in the energy transition if they are to meet the goals agreed upon in the Paris accord. One might therefore start to wonder if the mainstream approach focussing almost exclusively on solar and wind is way too narrow, and if we could not speed things up by choosing a strategy that allows for more different types of energy sources to be developed, built and implemented in parallel. Obviously if we were to broaden our approach we would also broaden the types of required resources, and therefore require less of those specific resources that we would otherwise require massive amounts of. This prevents bottlenecks in certain areas (such as the impending scarcity described in the previous paragraph) from slowing down the entire energy transition too much. To conclude, a broader approach to the energy transition equals a faster energy transition.
Additionally, an electricity grid that relies only on solar and wind is necessarily way more decentralized compared to our current grid consisting of relatively few centralized energy sources. Some authors present this as an advantage, after all this reduces our dependence on large electricity corporations. I say they are wrong, for starters because their argument is moot, the dependency on large electricity corporations is not removed, it is merely shifted towards large manufacturers and distributors of solar panels and wind turbines. Secondly, this creates a culture of ‘every man for his own electricity’, which is not only morally wrong from a Marxist perspective, it is also a bad idea from a technological perspective. Because an electricity grid is a complex thing where the amount of electricity sent into the grid must always be actively kept in balance with the amount of it that is used, if the balance is off too much a power outage is the result. This means that to prevent power outages, a constant flow of information is required between an enormous amount of sensors, switches, storage facilities and production facilities. This challenge would become exponentially more difficult the more decentralized and individualistic the electricity generation is.
An electricity grid is per definition a collective thing, completely decentralizing it would be as ill-advised as decentralizing the railways. It might work, but it would almost certainly be less stable and less robust than a centrally coordinated approach. In addition, it would be far from trivial to convert the existing electricity grid which has a more tree-like structure consisting of huge cables from the sources branching off into increasingly smaller cables, to the more web-like structure required for a more decentralized gird. Such a conversion, while technically possible, requires time, resources and effort, time which is running out and resources which might be better used elsewhere. Therefore, we would be better off opting for a strategy that requires less changes to the existing electricity grid, in other words a strategy that requires less decentralization.
Furthermore, let’s ask ourselves exactly why it is that we are in our current predicament. Obviously the answer is our current, almost exclusive, dependence on fossil fuels. The ‘exclusive’ part is very important here, in 2018 fossil fuels were still used for 70.2% of the electricity generation. Imagine if our starting point had been an electricity grid that was only for 50% dependent on fossil fuels where the remaining 50% was generated using something else. In this case it would of course be easier to remove the dependence on fossil fuels, than if 100% of the electricity would be generated using fossil fuels. The conclusion is that the larger the share of a certain energy source in the overall electricity generation, the harder it is to remove the dependence on it. What is the wise lesson to be learned here? It is that a diverse electricity grid is more flexible, and that therefore to avoid problems like this from becoming this huge in the future we should aim to create an electricity grid consisting of an as diverse as possible collection of energy sources.
An electricity grid based solely on solar and wind would in this aspect suffer from the exact same problem. If for whatever reason we would need to dial back the use of wind or solar we are once again in trouble. Or imagine what would happen if a massive volcano eruption covers all solar panels in an area spanning thousands of kilometres with a small layer of ash, reducing their efficiency by as much as 50%[12]. What if a huge storm (which are happening more frequently due to climate change) causes a large number of wind farms to temporarily be out of service. The critic will say that when the solar is out of service, the wind will take over, and when the wind is unavailable, the solar will take over. I would answer that this presumes that the other source is able to take over, which is not the case unless you either build a significant overcapacity of solar and wind, or create yet even more storage. As if we weren’t having enough trouble as is producing enough solar panels and wind turbines. I would propose an alternative solution: diversify! The more diverse the electricity grid is, the better it is able to cope with any one source being temporarily unavailable. Hence a diverse electricity grid is more robust.
An introduction to nuclear energy
A 2013 study concluded that nuclear energy has over the years prevented the emission of 64 gigatonnes of carbon-dioxide-equivalent (all greenhouse gasses taken together and expressed in terms of carbon dioxide), and in doing so has prevented 1.84 million air pollution-related deaths. In this number the authors have already subtracted the direct and indirect deaths from all nuclear disasters[13].

Figure 3: grams of carbon-dioxide-equivalent per kWh for different electricity sources
Furthermore, a 2014 study by the Intergovernmental Panel on Climate Change (IPCC) compared the total carbon-dioxide-equivalent released per kWh in the construction and during the life-time of different energy sources. A very relevant comparison because even during the construction of energy sources that are otherwise emission-free, fuel and electricity are used. The results of the study are shown in Figure 3 where we can see that nuclear energy scores comparable to wind energy with a median of about 12 grams of carbon-dioxide-equivalent per kWh. Solar energy (PV=photovoltaics) on the other hand scores four times worse with about 41 to 48 grams of carbon-dioxide-equivalent per kWh. As a side note, the same figure also shows us that biomass is not a solution, scoring only marginally better than coal. And that Carbon Capture (CCS) is also not a viable method to combat climate change still having a very large carbon-dioxide-equivalent footprint.
Dogmatically focussing exclusively on solar panels and wind turbines would be incredibly short-sighted. We would exchange an absolute dependency on fossil fuels with an absolute dependency on the weather, and would be required to completely redesign the existing electricity grid. We should instead focus on a more sustainable, faster and long-term approach, where we aim to create an electricity grid which is as diverse as possible. Don’t get me wrong, I’m very fond of solar and wind energy, but we cannot pretend that simply putting solar panels on our roofs, and wind turbines on our shores will solve our problem, as we have seen the problem is more complex and a strategy focussing exclusively on solar and wind would require massive investments in storage technologies and changes to the existing electricity grid. Nor should we presume that such a strategy would be fast enough to prevent climate catastrophe, or result in the best possible electricity grid. Therefore, I argue that we should diversify our approach, in order to speed up the energy transition, and to create a more stable, robust, and flexible electricity grid as a result. So in addition to solar and wind energy, we should also develop and deploy other (less known) non-fossil energy sources, such as: hydroelectricity, geothermal energy, tidal power, wave power, and yes nuclear energy. And as we shall see in parts 2 and 3, the latter is nothing to be scared of.
1. A. Richter, M. Hermle and S. W. Glunz, Reassessment of the Limiting Efficiency for Crystalline Silicon Solar Cells, in IEEE Journal of Photovoltaics, volume: 3 pages: 1184-1191, October 2013.
2. S. Rühle, Tabulated values of the Shockley–Queisser limit for single junction solar cells, in Solar Energy, volume: 130 pages: 139-147, June 2016
3. J.F. Geisz, R.M. France, K.L. Schulte, et al., Six-junction III–V solar cells with 47.1% conversion efficiency under 143 Suns concentration, in Nature Energy, volume: 5 pages: 326–335, April 2020
4. K. Bódis, I. Kougias, A. Jäger-Waldau, N. Taylor, and S. Szabó, A high-resolution geospatial assessment of the rooftop solar photovoltaic potential in the European Union, in Renewable and Sustainable Energy Reviews, volume: 114 pages: , October 2019
5. C. Bay Hasager, L. Rasmussen, A. Peña, L. E. Jensen, and P. Réthoré, Wind Farm Wake: The Horns Rev Photo Case, in Energies, volume: 6 pages: 696-716, February 2013
6. C. Bay Hasager, L. Rasmussen, A. Peña, L. E. Jensen, and P. Réthoré, Wind Farm Wake: The Horns Rev Photo Case, in Energies, volume: 6 pages: 696-716, February 2013
7. X. Han, D. Liu, C. Xu, W. Zhong Shen, Atmospheric stability and topography effects on wind turbine performance and wake properties in complex terrain, in Renewable Energy, volume: 126 pages: 640-651, October 2018
8. R. J. Barthelmie, and L. E. Jensen, Evaluation of wind farm efficiency and wind turbine wakes at the Nysted offshore wind farm, in Wind Energy, volume: 13 pages: 573–586, June 2010
9. C. Bay Hasager, L. Rasmussen, A. Peña, L. E. Jensen, and P. Réthoré, Wind Farm Wake: The Horns Rev Photo Case, in Energies, volume: 6 pages: 696-716, February 2013
10. N. G. Nygaard, and S. D. Hansen, Wake effects between two neighbouring windfarms, in Journal of Physics: Conference Series, volume: 753 pages: 032020, September 2016
11. F. Cebulla, J. Haas, J. Eichman, W. Nowak, and P. Mancarella, How much electrical energy storage do we need? A synthesis for the U.S., Europe, and Germany, in Journal of Cleaner Production, volume: 181 pages: 449-459, April 2018
12. E. Zorn, and T. R. Walter , Influence of volcanic tephra on photovoltaic (PV)-modules: an experimental study with application to the 2010 Eyjafjallajökull eruption, Iceland, in Journal of Applied Volcanology, volume: 5 pages: 2, January 2016
13. P.A. Kharecha and J.E. Hansen, Prevented Mortality and Greenhouse Gas Emissions from Historical and Projected Nuclear Power, in Environmental Science & Technology, volume: 47 pages: 4889−4895, 2013
Do you value this free article? Please consider a onetime donation or subsribe to read all of my content. Thank you!